Brief History: Reconnection
Table of Contents
Clicking on any marked section on the list below brings up a
file containing it and all unmarked sections immediately
following it on the list. This list is repeated at the beginning of each file.
- Introduction
- Discovery of the Radiation Belts
- Artificial Belts and Early Studies
- The Large Scale Structure
- Convection
- Reconnection
- The Open Magnetosphere
- Observational Tests
- The Polar Aurora
- Field Aligned Voltage Drops
- Birkeland Currents
- Substorms: Early Observations
- Substorms: The Satellite Era
- Substorms: Theory
- Convection in the Geotail
- Planetary Magnetospheres
- Other Areas
- Assessment
References: A-G
References: H-P
References: Q-Z
Back to "Exploration"
6. Reconnection
As early as 1942 cosmic ray detectors observed the arrival of high-energy ions associated with solar activity, reaching at times up to approximately 10 GeV [Forbush, 1946; Pomerantz, 1984; Van Allen, 1993]. For many years such events were credited to solar flares, although recent evidence points to a much better correlation with coronal mass ejections [Gosling, 1993]; their most plausible energy source, then as now, seems to be the intense magnetic field of sunspots. It was speculated that somehow part of that field was "annihilated" by a rapid process and its energy used to accelerate ions and electrons, the latter revealed indirectly by intense bursts of radio noise, and more recently, by X-rays.
The process most favored for such energy release was magnetic merging or magnetic reconnection (synonymous terms). It may be loosely defined as a flow of plasma in which some of the field lines threading the plasma pass through a neutral point or neutral line at which the magnetic field vanishes. The idea originated with Giovanelli [1947; Hones, 1984b] and was then developed by Sweet [1958] and especially by Dungey [1953, 1963, 1994, 1995; Stern, 1986].
Figure 6
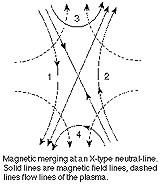
| The starting point is the observation that the field-line sharing property associated with equation (1) can be violated if plasma flows through a neutral point (Figure 6) where B=0 and where the field's direction is undetermined. In the X-type neutral point drawn here (actually a neutral line if this configuration extends unchanged into the third dimension) field lines cross in the pattern of the letter X and plasma arriving on field lines of the regions "1" and "2" depart on differently connected lines in "3" and "4". Dungey, Sweet and others proposed that this process might somehow modify the large-scale magnetic configuration and thereby release magnetic energy. Particles would be accelerated by the electric field associated with the motion, producing fast jets of plasma flowing away from the neutral line as the plasma exits on lines "3" and "4", and shocks which heat the plasma [Levy et al., 1964].
|
Additional effects must be invoked, for by (1), if E is finite and B tends to 0, the velocity v which particles need to keep up their field-line sharing property becomes infinite. Sweet [1950] showed that in conducting fluids with finite resistivity the plasma's motion lags behind that of field lines, and therefore reconnection theories have often assumed a finite (but small) resistivity in the region near B=0. Other processes which preclude an infinite v may also play a role, e.g. ion or electron inertia [Vasyliunas, 1975, table 2].
A somewhat different type of process ("group 2 merging" of Vasyliunas, [1975], p. 307) is illustrated by the collision of two bodies of plasma permeated by magnetic fields of equal intensity but opposing directions, separated by a "neutral sheet" of zero field intensity. The plasma may emerges as a narrow stream along the sheet, perpendicular to field lines, its magnetic field "annihilated" and its particles accelerated by the attendant E, though its electric neutrality may pose problems [Stern, 1990].
Magnetic reconnection is relevant to magnetospheric physics in two distinct ways: it makes possible a realignment of field line connections, e.g. the establishment of a linkage between the Earth's field and the IMF, and it may also release magnetic energy and accelerate particles. The first aspect is important to the concept of the open magnetosphere, the second to substorms, two items discussed separately further below.
As noted earlier, many researchers arrived at magnetospheric physics from the study of solar energetic particles, and they brought with them an interest in reconnection [Parker, 1963]. That led to a 1963 symposium at Goddard Space Flight Center [Hess, 1964] where, among other things, the theory of Petschek [1964, 1995] was presented, giving a more detailed scenario of the reconnection process. Important references to later work may be found in a comprehensive review by Vasyliunas [1975], in the proceedings of a 1984 conference at Los Alamos [Hones, 1984a] and in reviews by Sonnerup [1979] and by Forbes and Priest [1987]. Avenues explored nowadays include the relation to tearing instabilities in plasmas [Schindler and Birn, 1978], reconnection at multiple points [Lee and Fu, 1985] and relations to chaotic field line topology [Hesse and Schindler, 1988]. A growing number of studies simulate reconnection by means of fast computers.
There exists some evidence for reconnection from direct observations at the dayside magnetopause [e.g., Paschmann et al., 1979; Sonnerup et al., 1981] but it is difficult to verify details of the mechanism. In regions where reconnection seems likely to occur, magnetic fields are quite variable and with isolated spacecraft it is almost impossible to extract their structure. The existence of a rarefied "depletion layer" outside the magnetopause, seen only when the directions of B inside and outside are similar, is taken as evidence for reconnection. Claims have also been made that characteristic oscillations of the magnetic field observed near the dayside magnetopause, associated with southward IMF and termed "flux transfer events" [Russell and Elphic, 1978, 1979; Elphic, 1994], are local signatures of "patchy reconnection", but in spite of extensive studies, such events remain poorly understood.
7. The Open Magnetosphere
Reconnection was first applied to magnetospheric physics by Dungey [1961], as the key ingredient of his alternative theory of convection. Dungey proposed that an X-type neutral point (or line) at the front of the magnetosphere enabled terrestrial field lines to link up with interplanetary ones and produce "open" field lines, with one end on earth and the other in distant space.
| Figure 7
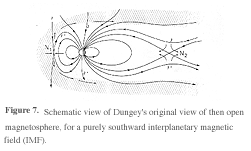
|
Figure 7 (from Hill [1983]) gives Dungey's original scenario, which assumed a purely southward directed interplanetary magnetic field (IMF). Lines 1 and 1' merge at a sunward X-type point (mp marks part of the magnetopause) to produce open lines 2 and 2', carried tailward by the solar wind in which they are embedded, to positions such as 3 and 3'. Ultimately these field lines reconnect at a distant neutral point in the tail, to produce an interplanetary field line 4 which is carried away by the solar wind, and a "closed" line 4' attached to Earth at both ends, which then flows sunward in the third dimension until it becomes the closed field line 1' which reconnects with 1. It is often held that these points are broadened to neutral lines of finite length in the direction perpendicular to the drawing, to accomodate the finite rate of flux reconnection.
The polar convection pattern and the polar electric field resulting from this motion qualitatively resemble those expected from the viscous-like drag proposed by Axford and Hines, The magnetopause now is no longer a surface containing field lines, but instead is often identified with an observed sharp discontinuity in the magnetic field, interpreted theoretically as a shock transition related to magnetic reconnection. It will have a normal magnetic component Bn which, by all predictions, is quite small (about 0.5-1 nT), making it difficult to confirm or refute this scenario by in-situ magnetic observations.
Figure 8
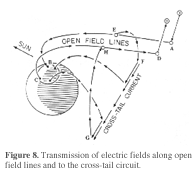
| Reconnection at N1 probably imparts little energy to the plasma. Its real significance is the creation of "open" field lines such as 2 and 2', linked to both the solar wind and the ionosphere. Because electric currents in a plasma flow easily along field lines, such lines can form a dynamo circuit, a closed circuit part of which traverses a medium moving relative to the rest. A circuit of this kind (ABCD in Figure 8) can drive an electric current and produce an electric field in the polar ionosphere: its energy is obtained by slowing down the moving solar wind or mantle plasma threaded by it, and much of that energy is then deposited as ohmic heat in the ionospheric part of the circuit.
|
Note that the currents in this circuit (in both polar caps) flow earthward on the morning side of the pole (AB) and away from Earth on the evening side (CD), which is also the pattern of region 1 Birkeland currents (below). If the tail current follows the "theta pattern" of Axford et al. [1965; Dessler and Juday, 1965], the circuit EFGH may also be viewed as a dynamo, supplying energy that heats the plasma sheet.
8. Observational Tests
Dungey's process is expected to operate best if the IMF is purely southward, for then the IMF direction matches that of the Earth's polar field lines which link up with it (Figure 9a). If the Bz component of the IMF is southward (negative) but additional components also exist, the situation is known as "southward IMF": the linkage is still relatively easy, but interplanetary field lines must bend somewhat to make the connection (Figure 9b). The bending becomes severe if Bz > 0 ("northward IMF"), because interplanetary field lines then start out headed for the "wrong" pole (Figure 9c).
| Figure 9
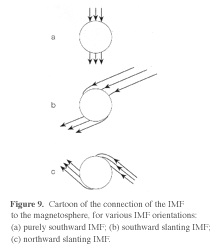
|
A major boost for Dungey's ideas was the discovery [Fairfield, 1966, 1967] that the level of magnetospheric "storminess" and of energy transfer from the solar wind to the magnetosphere depended strongly on IMF Bz. During southward IMF substorms are more frequent, the polar caps (assumed to contain open field lines) are fairly large and display a well-defined 2-cell convection pattern, and auroral ("Birkeland") currents (further below) are steady and strong. At times of northward IMF the magnetosphere is much quieter, the polar caps shrink and their E weakens. The effect is asymmetrical: when Bz is southward, increases in its magnitudes are correlated with increased activity, but the magnitude of a northward Bz seems to matter little [Burton et al., 1975]. A strong dependence of magnetospheric behavior on IMF Bz was later demonstrated by numerical simulations of the magnetosphere, conducted on fast computers and based on the MHD equations [e.g., Walker et al., 1993].
The Svalgaard effect [Svalgaard, 1968, 1972, 1973; Mansurov, 1969; Wilcox, 1972] is another interesting piece of evidence for a linkage between the IMF and terrestrial field lines. That is an asymmetry in the daily variation in polar regions, correlated with the interplanetary By component.
Owing to the interplay between solar wind outflow and the Sun's rotation, IMF field lines near Earth tend to lie close to the (x,y) plane, with B in the (x,-y) and (-x,y) quadrants and making an angle of about 450 with the x-axis, as predicted by Parker. Such lines can have one of two polarities--away from the Sun or towards it, corresponding to positive or negative By. Wilcox and Ness [1965; Wilcox, 1972] studied the prevalence of such polarities and showed that they tended to persist over times of a week or two, suggesting that the IMF in the plane of the ecliptic formed large-scale "sectors" of outward-pointing or inward-pointing field lines, co-rotating with the Sun. Often only two sectors exist, but at times they are more numerous, depending on the distribution of magnetic field sources on the Sun.
In 1926 K. Lassen established a magnetic observatory on Greenland which among other things observed the local daily magnetic variation. Around 1968 Svalgaard noted that the variation on quiet days could be classified as belonging to one of two patterns, and Wilcox suspected these correlated with interplanetary sectors. A large "blind" test was conducted [Friis-Christensen et al., 1971] and it confirmed the effect. The phenomenon might be connected with the By-related asymmetry in the pattern of polar E, later found by Heppner [1972b] ; it would be hard to explain, if terrestrial field lines had no link to the IMF.
Another observation suggesting the existence of "open" field lines is the asymmetric access to the polar caps of the high-energy tail ("strahl") of solar wind electrons (» 0.5 keV), producing the "polar rain" precipitation. Depending on the IMF sector in which the Earth is immersed, that "rain" is much more intense in the polar cap whose magnetic polarity allows direct connection to the Sun [Yaeger and Frank, 1976].
Problems in observing merging near N1 were already described. The distant nightside neutral line N2 has never been clearly identified: its signature should be a reversal of Bz from northward (Bz>0) to southward (Bz<0). Around 1983, when ISEE-3 probed the distant tail, it observed that periods of Bz<0 became more frequent at distances greater than approximately130 RE [Slavin et al., 1985], but that was only a statistical average of a rather variable quantity.
ISEE-3 has also shown [Slavin et al., 1985] and Geotail has confirmed, that in the distant tail past about 100 RE , plasma flowed tailwards at velocities that tended to increase with distance, up to where they about matched the velocity of the solar wind. This might be due to viscous transfer of plasma and momentum, but could also be the result of reconnection.
|